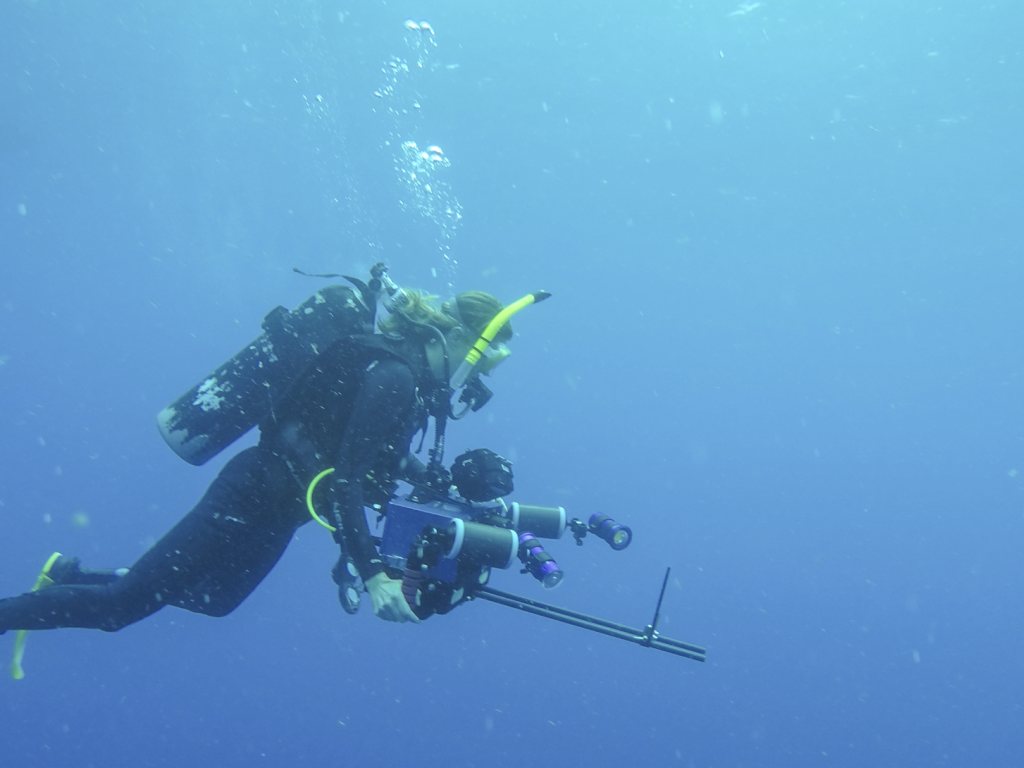
Editor's note: This article was produced by a student participating in the course J477/577: Strategic Science Communication, a collaboration between the School of Journalism and Communication’s Science Communication Minor program and the Research Communications unit in the Office of the Vice President for Research and Innovation.
While offshore scuba diving, University of Oregon researchers collected different species of salps, a type of transparent, gelatinous plankton, that can teach us how to move more efficiently in different parts of the ocean. These samples showed the different arrangements or architectures that various slap colonies form while developing, which can inform better bioengineering designs. Kelly Sutherland, an associate professor, and Alejandro Damián-Serrano, adjunct professor, both at the Oregon Institute of Marine Biology, collected and photographed the 48 different salp species to create a developmental framework for colonial architectures to aid future research. Their developmental framework was published in The Biological Bulletin.
“Salps are the cow equivalent of the ocean,” Damián-Serrano said. “They graze by filter-feeding in the open ocean.”
Some salp species are the size of a jellybean, while others can be compared to a mini sweet pepper, but all salp species experience the same beginning. There are two stages in a salp’s life cycle: solitary and a colonial chain. A solitary salp asexually creates a chain of genetically identical salps. Salp chains start as a coil in a solitary salp’s gut and eventually break free as the chain grows.
As the chains develop, they change form and orientation based on the species because individual salps move around and shift their location. Salp colonial architectures include transversal chains, oblique chains, linear chains, whorls, clusters, and helical solenoids. While these arrangements are visually interesting, they also play an important role in how salp colonies move through the ocean.
Solitary salps glide around by jet propulsion because of two siphons located on their barrel-shaped bodies that are designed to suck water in and shoot it back out of their rear end. Similarly, salp colonies navigate the oceans with multi-jet propulsion. This allows each salp to swim at its own asynchronous pace, but the colony synchronizes its swimming when threatened by predators such as sea turtles or strong waves. These chains allow colonies to experience the benefits of drag reduction and steady swimming. Salp colonies travel from the ocean depths to the surface every night to feed under the cover of darkness and then return to the deep before the sun rises. This long-distance movement is the fastest and most energy-efficient by salp colonies in a linear chain arrangement.
“This helps explain their success at migrating vertically,” Sutherland said. “And if we can understand this then we can apply this knowledge in another space.”
Engineering that mimics nature
Engineers could take design elements from different salp colonies to improve underwater vehicles so people could more safely explore remote parts of the ocean. Knowing which architectures are most efficient underwater allows engineers to consider propeller arrangements to optimize performance. Researchers found that while linear salp arrangements are fast, cluster arrangements offer more maneuverability advantages, and knowing the different benefits of each arrangement is important for improving these tools.
This knowledge could also be applied to underwater robots that could mimic this design to better explore tight spaces in the ocean. “Imagine squeezing through cracks or being able to bend and change trajectory,” Damián-Serrano said. “This technology does not exist yet, but we have all the elements to make it happen.”
Creating underwater robots that mimic salp colonies has several advantages. For example, imagine a salp robot collecting data around a coral reef. If part of the chain broke off on the reef, there would still be some units to report the data, so there would be less loss since the data is distributed across multiple units, Sutherland said.
To work toward these bioengineering improvements, a standardized framework and terminology are important for effectively communicating and advancing this research.
“If we do not have a framework for something then we can't talk about it,” Sutherland said. “By creating this language and set of terms we can take our research a step further.”
— By Kirstin Saha, for the Office of the Vice President for Research and Innovation