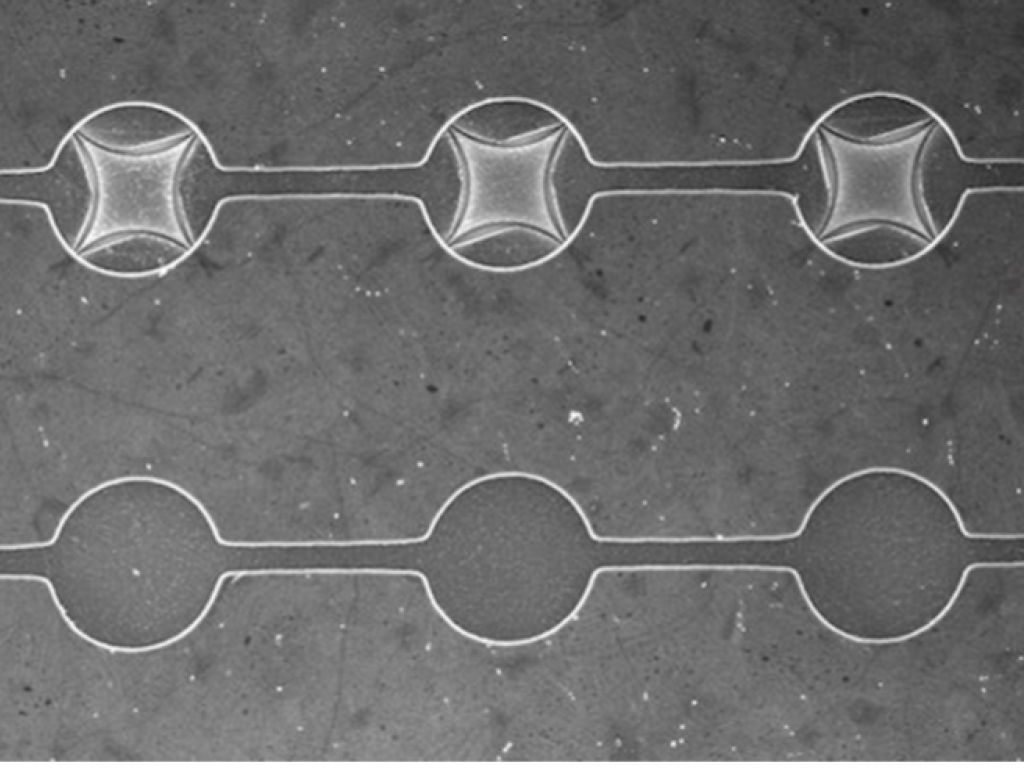
Editor's note: This article was produced by a student participating in the course J477/577: Strategic Science Communication, a collaboration between the School of Journalism and Communication’s Science Communication Minor program and the Research Communications unit in the Office of the Vice President for Research and Innovation.
To an outside eye, the research conducted by Benjamín Alemán, associate professor of physics at the University of Oregon, seems to be pulled straight from a science fiction story: Creating drums that “hear” light, manipulating nanoscopic technology with laser beams, and creating programmable materials with properties unlike anything else in nature. In the Alemán lab, innovations on a nanoscopic scale will hopefully help revolutionize the larger world of engineering and computing.
Alemán’s current project involves designing a lattice of extremely small drumheads, called nanomechanical resonators, whose properties can be manipulated in real time. Each drumhead, just one atom thick, vibrates at a specific frequency and can influence the vibrations of adjacent drumheads.
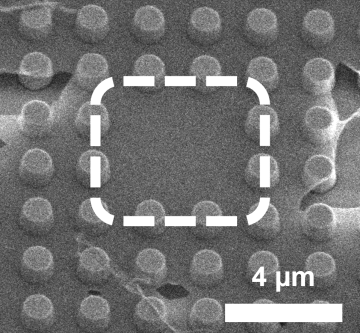
“Imagine this is like a really big guitar with a thousand strings on it, where you can tune each individual string to any frequency you want,” Alemán said. “And then you can tune how much the strings can talk to each other too. You can turn it off completely so they don’t talk to each other at all, or you can crank it up.”
When these drumheads are tied together in a grid, the resulting interface can be used to process information far more efficiently than current computing technology. Rather than classical computers, which store data in binary units of 1 or 0, these resonators store data in the value of their vibrating frequency, as well as what phase of vibration it is in at a given point in time. While a binary system allows each node to have 2 possible outcomes, these continuous variables result in many more possible identities for a single drumhead, resulting in more detailed information stored in a small unit. This project is ambitious, but the Alemán lab has been confidently tackling it one piece at a time.
“We’re building up this platform, one tool at a time, and we’re one step away,” Alemán said. “We have to be able to completely turn off the coupling between adjacent little drumheads, and then turn the coupling up to high levels. That’s the last step we have and it’s going to be probably the most interesting.”
Future implementation and applications
The resulting programmable interface could be incredibly versatile. In addition to being used for highly efficient computers, it could be used by physicists to simulate the behavior of different natural materials and phenomena, and even by engineers to create powerful cooling mechanisms. The list of possible applications goes on, which is one of the highlights of being involved in nanoscale physics research.
“It’s pretty exciting to see something new and then think, ‘OK that’s cool from a science perspective,’ and then also ‘What could that be used for that’s useful for humans?’” Alemán said.
In this field, creativity makes the difference between a problem and an innovation. It’s not uncommon for scientists to stumble upon discoveries that can provide solutions to problems they hadn’t even considered when conducting the research. One of the lab’s recent inventions, a fast, highly sensitive light detector, is unlocking opportunities for astronomical research and thermal imaging technology.
“Yeah, that was kind of an accident, really,” Alemán said. “One big happy accident. The effect that was underneath the technology was really a nuisance to us.”
For a long time, an obstacle in Alemán’s work was the resonators’ sensitivity to light. Even small amounts of light in the room would alter the frequency of the resonator, much like how the pitch of a musical instrument can be affected by heat. Eventually, the lab decided to see if they could use this light sensitivity for a different purpose. A few years later, they secured a patent on a light detector that is nearly a million times faster and a million times more sensitive than modern commercial thermal imaging technology. This instrument could be useful for research institutes like NASA, to identify hard to spot objects in our solar system and beyond, as well as for firefighters who need accurate thermal imaging to see through thick smoke and monitor the spread of fire.
Overcoming obstacles
Working with materials at such a small scale requires a lot of precision, and there are many potential disruptors that have to be accounted for. The lab space itself is filled with an intricate arrangement of lenses and mirrors, which shape the laser beams that are focused onto the samples. Alemán said each piece serves a purpose. The placement of one requires considering the positions of all the other pieces in the system. The smallest of disruptions will interfere with the positioning of the sample in relation to the light and produce undesired effects, but mistakes are part of the process.
“It’s like, ’Oh gosh, OK, that’s hard. How are we going to get through that?’ And then take a step back and think about creative solutions,” Alemán said. “Creativity, excitement, and enthusiasm are always the most important things. The motivation to get up and do it are always the most important things in any field, and that’s no different here.”